Numerical Investigation on the Pressure Drag of Some Low-Speed Airfoils for UAV Application
DOI:
https://doi.org/10.37934/cfdl.13.2.2948Abstract
Progressive advancements in small to medium-sized fixed-wing UAVs call for prototype designing to be fast, accurate, and economical. This requires the numerical assessment of airfoil performance to be based on high fidelity replication of wind tunnel data. Furthermore, integration of drag reduction techniques is attractive as improvements in endurance, payload capacity and reduction in carbon footprints can be attained. Since a variety of suitable airfoil geometries are currently available for this application, selecting a fitting candidate can be difficult therefore risking potential gain in efficiency. The aim of this paper is to assist in resolving this issue by investigating the variation in pressure drag and its distribution with respect to the type of airfoil geometry, angle of attack, and the contribution of pressure towards the total drag at low Reynolds numbers. The airfoils selected in this study comprises of the NACA 4415, FX 61-184, E420 and S1223 which are preferred for subsonic UAV applications in addition to having the NACA 0012 serving as a standard profile. Performance of the S1223 airfoil was examined at a chord-based Reynolds number of 0.3 x 106 with the remaining airfoils at 1.0 x 106 for a range of angle of attack of around 0-10°. The unsteady 3-equation Intermittency SST model from ANSYS FLUENT 2020 was utilized with gradual reduction of timestep from 0.001s, 0.0005s, 0.00025s and 0.0001s. Experimental lift and drag validation across the airfoils generally suggest that the transitional model regularly outperforms XFOIL. Among the selection, concave airfoils such as the E420 and S1223 excel in delivering high lift at the expense of an increase in drag. Evaluation of the l/d ratio alone may underestimate their potential. Hence, further studies should focus on the implementation of drag reduction techniques on concave airfoils to enhance their performance. At the maximum tested angle of attack, the E420 reaches a cl value of 2.09 and S1223 at 1.98 while the FX 61-184 only at 1.57 and NACA 4415 at 1.36.
References
Eisenbeiss, Henri. "A mini unmanned aerial vehicle (UAV): system overview and image acquisition." International Archives of Photogrammetry. Remote Sensing and Spatial Information Sciences 36, no. 5/W1 (2004): 1-7.
Shakhatreh, Hazim, Ahmad H. Sawalmeh, Ala Al-Fuqaha, Zuochao Dou, Eyad Almaita, Issa Khalil, Noor Shamsiah Othman, Abdallah Khreishah, and Mohsen Guizani. "Unmanned aerial vehicles (UAVs): A survey on civil applications and key research challenges." Ieee Access 7 (2019): 48572-48634. https://doi.org/10.1109/ACCESS.2019.2909530
Marqués, Pascual, and Andrea Da Ronch. Advanced UAV Aerodynamics, Flight Stability and Control. Wiley, 2017. https://doi.org/10.1002/9781118928691
Nagel, Alexander, and Misha Shepshelovich. "The concept of high-lift, mild stall wing." In 24th International Congress of the Aeronautical Sciences, Yohogama, Japan, 29August-03 September. 2004.
Selig, Michael S., and James J. Guglielmo. "High-lift low Reynolds number airfoil design." Journal of aircraft 34, no. 1 (1997): 72-79. https://doi.org/10.2514/2.2137
Basri, Adi Azriff, Ernnie Illyani Basri, Mohd Firdaus Abas, Faizal Mustapha, Mohamed Thariq Hameed Sultan, and Kamarul Arifin Ahmad. "UAV NACA4415 wing structural performance analysis subjected to external aerodynamic load using Schrenk’s approximation." Journal of Advanced Research in Fluid Mechanics and Thermal Sciences 60, no. 2 (2019): 178-190.
Althaus, Dieter. Experimental results from the Laminar Wind Tunnel of the Institut für Aero-und Gasdynamik der Universität Stuttgart. Vol. 1. F. Vieweg, 1981.
Eppler, Richard. Airfoil design and data. Springer Science & Business Media, 2012.
Windte, Jan, Rolf Radespiel, Ulrich Scholz, and Bernhard Eisfeld. RANS simulation of the transitional flow around airfoils at low Reynolds Numbers for steady and unsteady onset conditions. Technical Univ Braunschweig (Germany) Inst Of Fluid Mechanics, 2004.
Langtry, Robin Blair, F. R. Menter, S. R. Likki, Y. B. Suzen, P. G. Huang, and S. Völker. "A correlation-based transition model using local variables—part II: test cases and industrial applications." (2006): 423-434. https://doi.org/10.1115/1.2184353
Khayatzadeh, Peyman, and Siva Nadarajah. "Laminar?turbulent flow simulation for wind turbine profiles using the ?–transition model." Wind Energy 17, no. 6 (2014): 901-918. https://doi.org/10.1002/we.1606
Lanzafame, R., S. Mauro, and M. Messina. "Wind turbine CFD modeling using a correlation-based transitional model." Renewable Energy 52 (2013): 31-39. https://doi.org/10.1016/j.renene.2012.10.007
Inc ANSYS, ANSYS Fluent Theory Guide (release 2020 R2). 2020, Ansys Canonsburg, PA.
Menter, Florian R., Robin Blair Langtry, S. R. Likki, Y. B. Suzen, P. G. Huang, and S. Völker. "A correlation-based transition model using local variables—part I: model formulation." (2006): 413-422. https://doi.org/10.1115/1.2184352
Langtry, Robin Blair. "A correlation-based transition model using local variables for unstructured parallelized CFD codes." (2006).
Keating, Mark, and A. N. S. Y. S. Principal Engineer. "Accelerating CFD solutions." advantage 1 (2011): 48.
Drela, Mark. "XFOIL: An analysis and design system for low Reynolds number airfoils." In Low Reynolds number aerodynamics, Springer, (1989): 1-12. https://doi.org/10.1007/978-3-642-84010-4_1
Selig, Michael S. Summary of low speed airfoil data. SOARTECH publications, 1995.
Sheldahl, R. E., and P. C. Klimas. "Aerodynamic characteristics of seven airfoil sections through 180 degrees of attack for use in aerodynamic analysis of vertical axis wind turbines, SAND80-2114." Sandia National Laboratories, Albuquerque, NM (1981). https://doi.org/10.2172/6548367
Miley, Stan J. Catalog of low-Reynolds-number airfoil data for wind-turbine applications. No. RFP-3387. Rockwell International Corp., Golden, CO (USA). Rocky Flats Plant; Texas A and M Univ., College Station (USA). Dept. of Aerospace Engineering, 1982. https://doi.org/10.2172/5044823
Wortmann, F. X. "A critical review of the physical aspects of airfoil design at low Mach numbers." NASA CR 2315 (1973): 179-196.
Mangalam, S., J. R. Dagenhart, J. Meyers, and T. Hepner. "The Görtler instability on an airfoil." In 23rd Aerospace Sciences Meeting, p. 491. 1985. https://doi.org/10.2514/6.1985-491
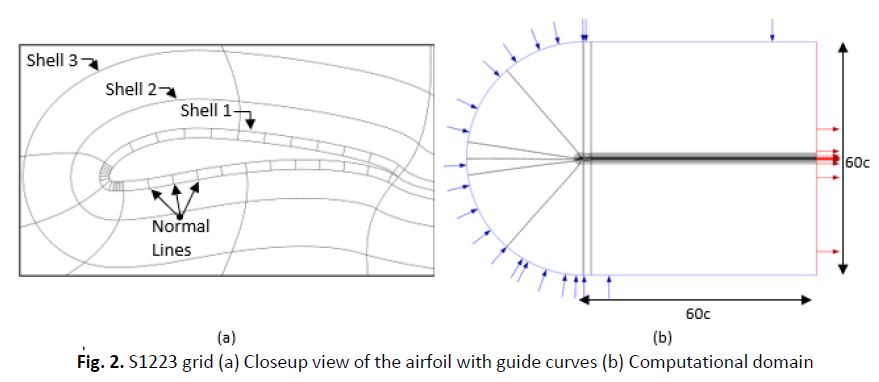